Waveguide and light extraction
The operation of Quantum Cascade Lasers (QCLs) has been demonstrated over a broad frequency range of the Terahertz spectrum, from 5.3THz to 1.2THz [1,2,3]. Most potential applications of THz QCLs require a source that exhibits an excellent spatial and spectral control of the radiated emission. To date the best performance for THz QCLs, in terms of operating temperature and frequency coverage, have been obtained with the so called double metal waveguides (Schematic in Figure 1a) [4]. These are very similar to microwave microstrip transmission lines: the active region is sandwiched between two metal layers providing a strong confinement of the optical mode in the gain medium. However, the subwavelength cross section of the waveguide, as well as the interferences from the front and back facets, cause highly non-directional far-field beam pattern and poor slope efficiency for these structures.
A strong interest is then dedicated to the design of a suitable waveguide to improve the extraction efficiency and the laser beam quality, while keeping the advantages of the double metal waveguide. We have investigated several approaches: an adjunction of a horn antenna at the facet of a double metal waveguide structure [9], an integrated patch array antenna [10], and a Vivaldi antenna [11].
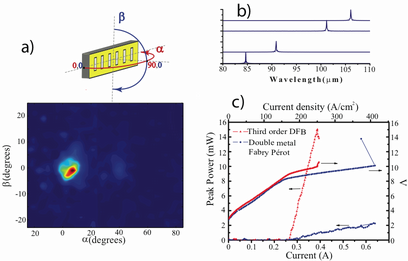
The first is based on the fabrication of mode matching devices such as horn antennas. It has shown promising results (Figure 1) but it requires challenging technological efforts and in addition do not provide any spectral control of the laser emission.
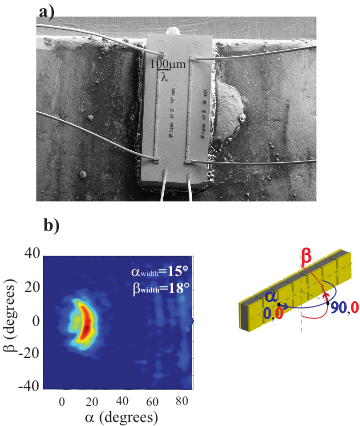
Another approach is based on the design of distributed feedback waveguides which features a grating resonant with the third order Bragg condition [5]. We show that an improvement of the extraction efficiency results in control of the laser emission wavelength and enhanced output power. Moreover, the grating can act as an array of phased linear sources [6], reshaping the typical wide and patterned far-field of double metal waveguides into a narrow beam of ~10° divergence in both directions, emitted along the direction of the device length (Figure 2). Moreover, thanks to the peculiar phase relation between the sources of this array, we demonstrate good beam quality even in the case of waveguides with sub-wavelength lateral dimensions (photonic-wire lasers). Each emitter is in fact shifted by pi with respect to the neighboring one, unlike the case of surface emitting grating, and it is placed at a distance of lambda/2 from them. Thanks to this approach it is possible to design a waveguide that is not limited by the standard diffraction limit for the emission divergence in the lateral direction (Figure 3) [7].
In a sub-wavelength device the mode is leaking out from the guiding cavity and is thus sensitive to the refractive index changes of the surrounding medium. This effect was used to tune the resonant frequency of the guided mode by means of nitrogen gas condensation for 20 GHz [8].
The approach with the patch array antenna (see Fig. 1), integrated on BCB polymer, has been optimized for surface emission of single mode lasers at specific wavelengths, interesting for astrophysical applications. For more details, visit the section QCLs for Astrophysical Applications.
Vivaldi antenna
A major drawback of double metal waveguide THz QCLs has been their poor far-field properties. We developed a broadband antipodal Vivaldi antenna [11], fabricated with a double-sided photolithography, metallization and lift-off process on a low-loss transparent polymer, as shown in Fig. 4.(b). The final antenna structure was aligned and positioned in front of a double metal waveguide THz QCL, as illustrated in Fig. 4.(a). Once the optical mode propagating inside the waveguide reaches the end facet, it remains confined between the two antenna flares. The antenna’s exponentially curved flare profile results in an adiabatic in-plane mode expansion, producing a narrow beam measured far-field pattern (23°x19°) with octave-spanning bandwidth, see Fig. 4.(c,d). The antenna also acts as a wave retarder, rotating the polarization from vertical towards horizontal polarization. The radiation mechanism is not based on a narrow resonance and is inherently broadband (designed for frequencies between 1.5-4.5 THz). Besides the significantly improved far-field of broadband devices, a major advantage of this approach is that the antenna does not affect the laser’s emission spectrum and current-voltage characteristics, as well as frequency comb operation, typically fragile to modifications of the waveguide structure.
![Figure 4. (a) Vivaldi antenna illustration. (b) Microscope image of a fabricated device. Measured (c) and simulated (d) far-field. (e) Measured emission spectrum. (f) Beatnote map, indicating frequency comb operation. Figure adapted from Ref. [10].](/research/thz-lasers-and-technology/waveguide-light-extraction/_jcr_content/par/fullwidthimage_100955628/image.imageformat.930.1179748129.png)
References
[1] Kohler, R., et al. Terahertz semiconductor-heterostructure laser. Nature 417, 156–159 (2002) - external page DOI
[2] Williams, B.S. Terahertz quantum-cascade lasers. Nature Photonics 1, 517-525 (2007) - external page DOI
[3] Scalari, G., et al. THz and sub-THz quantum cascade lasers . Laser and Photon. Rev. 3 , 45-66 (2009) - external page DOI
[4] Belkin, M.A., et al. Terahertz quantum cascade lasers with copper metal-metal waveguides operating up to 178K. Opt. Express 16, 3242-3248 (2008) - external page DOI
[5] M.I. Amanti, M. Fischer, G. Scalari, M. Beck and J. Faist, “Low-divergence single-mode terahertz quantum cascade laser,” Nature Photonics 3, 586-590 (2009) - external page DOI
[6] C.A. Balanis, Antenna Theory (Wiley – Interscience, 2005)
[7] M I. Amanti, G. Scalari, F. Castellano, M. Beck, and J. Faist, "Low divergence Terahertz photonic-wire laser," Opt. Express 18, 6390-6395 (2010) - external page DOI
[8] D. Turcinkova, M.I. Amanti, F. Castellano, M. Beck and J. Faist, Appl. Phys. Lett. 102, 181113 (2013) - external page DOI
[9] M.I. Amanti, M. Fischer, C. Walther, G. Scalari and J. Faist, "Horn antennas for terahertz quantum cascade lasers." Elect. Lett. 43, 450 (2007) - external page DOI
[10] C. Bonzon, I. C. Benea Chelmus, K. Ohtani, M. Geiser, M. Beck and J. Faist, "Integrated patch and slot array antenna for terahertz quantum cascade lasers at 4.7 THz." Appl. Phys. Lett. 104, 161102 (2014) - external page DOI
[11] Senica, U., et al., “An antipodal Vivaldi antenna for improved far-field properties and polarization manipulation of broadband terahertz quantum cascade lasers,” Appl. Phys. Lett. 116, 161105 (2020) - external page DOI