Nonlinear optical properties of THz quantum cascade structures
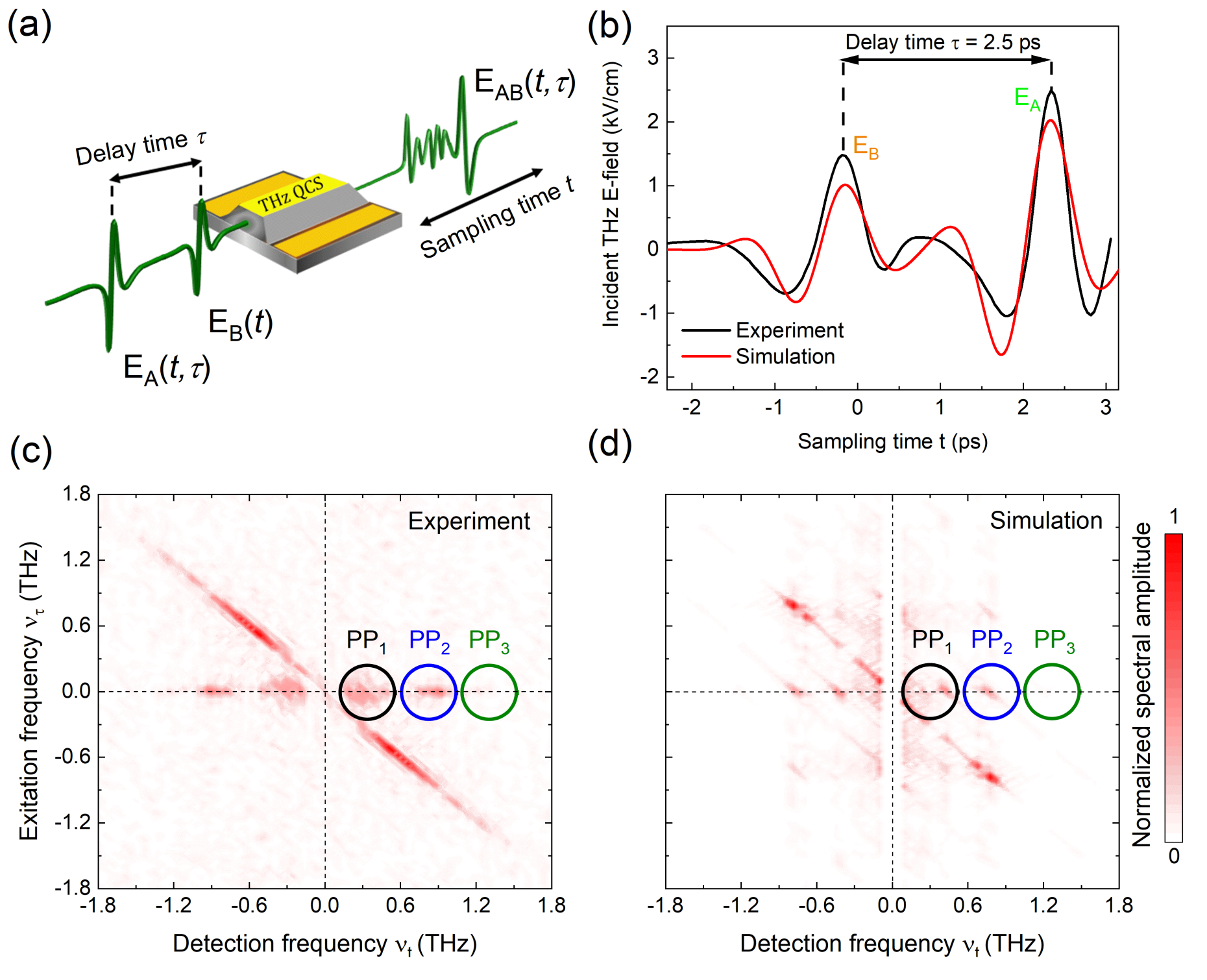
An important development for spectroscopy applications is to increase the spectral coverage of frequency combs, as well as to limit their noise. This requires understanding of the frequency comb formation mechanisms, of which there are two main contirbutors with different physical origin. The first is associated with spatial hole burning (SHB) in a Fabry-Perot (FP) cavity and the second relies on four-wave mixing (FWM). The former process can be seen as an effective non-linear response of the whole cavity, while the latter arises from the third-order susceptibility 𝜒(3) of the gain material itself. Hence, it is of vital importance to study the nonlinear properties of THz quantum cascade structures and optimize them with respect to high orders of the nonlinear susceptibility. Here, a time resolved system study allows for detailed information about the interplay of the material quantum levels and externally applied optical fields over a broad spectral range and for all nonlinear orders in the light-matter interaction.
Therefore, we employ a two-dimensional THz time-resolve spectroscopy (Fig. 1 (a)) to reveal the time-resolved nonlinear optical response of a THz quantum cascade structure (QCS) [1]. The investigated QCS possess a low frequency active region design with lasing frequency at 1.2 THz as shown in Ref. [2]. The nonlinear response at zero bias of this structure shows several pump-probe signals of third order (so called pump-probe signals), which are color coded with circles in Fig.1 (c)-(d). From the time-resolved evolution of each pump-probe signal one can extract the system dynamics and hence the coherent and in-coherent carrier evolution. Besides the experimental study, a time-resolved density-matrix model [3] based on the master equation in Lindblad form (which ensures the positivity of the density matrix and includes coherent and incoherent transitions between all density-matrix elements) is used to simulate the experiment, and gives direct access to the time-evolution of all density matrix elements. This model enables to explain the experimental observations, which only measures directly the electric field induced by the active material, in terms of the time-varying occupations and coherences of the underlying quantum system. Moreover, the developed model offers an additional benefit, such as distinguishing the scattering mechanism and their influence on the system dynamics. This allows for assessment and improvement of specific nonlinear properties, and the development of novel electro-optical devices with giant nonlinearities, such as saturable absorbers, pulse shapers, frequency converters, or frequency comb sources.
References
[1] S. Markmann, M. Franckié, S. Pal, D. Stark, M. Beck, M. Fiebig, G. Scalari and J. Faist, ”Two-dimensional spectroscopy on a THz quantum cascade structure” Nanophotonics (2020), external page DOI
[2] C. Walther, M. Fischer, G. Scalari, R. Terazzi, N. Hoyler, and J. Faist, ”Quantum cascade lasers operating from 1.2 to 1.6 THz“ Appl. Phys. Lett. 91 131122 (2007), external page DOI
[3] G. Kirsanskas, M. Franckié, and A. Wacker, ”Phenomenological position and energy resolving Lindblad approach to quantum kinetics” Phys. Rev. B 97, 035432 (2018), external page DOI